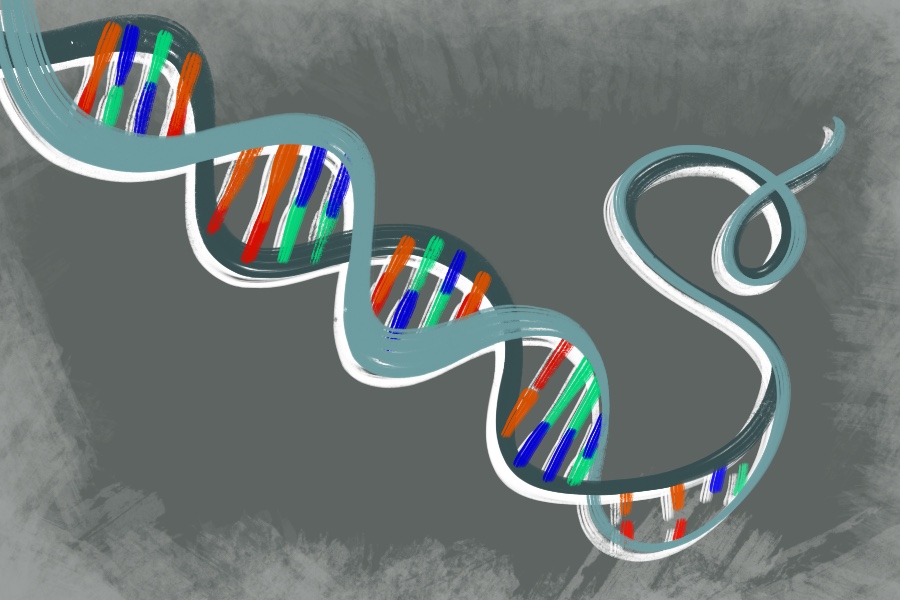
Computational modeling reinforces biological hypothesis of DNA-unlinking mechanisms
A team of international scientists have provided compelling evidence of how complex chains of bacterial DNA can unwind from interlinked states. A paper in Scientific Reports compiled the student’s findings, which build on previous work performed on the bacteria E. coli by this team in Japan, the United Kingdom and the United States over the last decade.
Biologists assumed there existed a unique pathway which methodically unlinked knots one by one to separate replicated strands of genetic material in the minimal number of steps. More evidence was needed to back up these assumptions, and mathematical data from this research team supports the shortest possible pathway hypothesis.
E. coli is a model organism used in scientific experiments due to its quick generation time and ease of use. Scientists have mapped the entire genome of the bacteria. Although DNA differs greatly in size and structure between living organisms, cells share remarkably similar processes and machinery to uncoil knotted strands. These similarities allow the results of the experiment to transcend beyond the mechanisms E. coli uses to unlink DNA.
“When you look at the bacteria E. coli, the bacterial chromosome is a circle — big and all crunched up inside the cell, but it’s a circle,” said Mariel Vazquez, the senior author of the paper and a professor of both mathematics and microbiology and molecular genetics at UC Davis. “Before the cell divides into two cells, this circle needs to be copied.”
DNA exists in a coiled state within living cells. During replication, DNA is copied by certain enzymes before the cell can divide, which leaves each daughter cell with genetic instructions for normal processes.
“As a cell replicates its DNA, the cell volume increases in proportion, so at least roughly the concentration of DNA in a cell is always the same,” said David Sherratt, a professor of biochemistry at the University of Oxford and one of the authors of the paper.
Molecular machinery unzips portions of the chain to expose the code to be transcribed, which builds up tension. Complicated knots are relaxed downstream by other enzymes, but the copied DNA structures are interconnected and need further coaxing to be separated.
“Instead of having two newly replicated circles that are separate, so that each one of them can go to separate cells, they are interlinked in very complicated ways,” Vazquez said.
The long chains of DNA have multiple enzymatic methods of unlinking. One process uses enzymes called topoisomerases, which cut the backbone of one or more DNA molecules and feed a strand through the break to reduce supercoiling. In another process, an enzyme called recombinase chops both strands at a specific site, unwinds them and reseals the break.
“The two strands of duplex DNA are interlinked — one link per 10 base pairs,” Sherratt said. “This means to copy the E. coli chromosome, around 450,000 links need to be reduced — every single one if the newly replicated sisters are to separate from each other!”
To simulate the genetic knot complexes, the team created models representing DNA chains with possible sites where enzymes could snip and reconnect the chains. Hundreds of different spatial orientations, or topologies, were considered and millions of possibilities were simulated. Vast computing power was necessary in order to calculate the probabilities of the different unlinking pathways to determine which was most likely under certain mathematical assumptions.
“We were very fortunate to have access to a new cluster that we built at UC Davis fairly recently, called CRICK,” said Robert Stolz, a third-year Ph.D. candidate in integrated genomics and genetics at UC Davis and the paper’s first author.
After organizing the data and investigating the probabilistic pathways of DNA unlinking, the interdisciplinary team compiled evidence to support the hypothesis of the biologists.
“What happens is the that the unique shortest pathway identified under the strongest assumption is most likely,” Stolz said.
The team focused on macroscopic conformations of DNA strands for their analysis. Modeling each base pair of a DNA strand, even relatively small ones, and then simulating knot topologies is beyond the current computing power of researchers.
“I am most interested in studying DNA structure — not at a very high-resolution level, but rather at the large scale,” Vazquez said. “When you look at the DNA molecule, there is an extremely long chain that fits in a very small environment. What is the geometry that this chain adopts?”
Knot theory is useful in understanding how DNA can unwind in living systems, but also generates interest in diverse fields such as engineering and astronomy. Smoke rings, liquid phenomena and the flashes of solar corona exhibit similar reconnection characteristics as DNA.
“It turns out that the work that we’re doing is of a lot of interest to people doing fluid dynamics because the same sort of networks of different topologies that we observe in our study also emerge in other systems,” Stolz said.
Written By: George Ugartemendia — science@theaggie.org